Introduction
Hydrogels are soft and wet materials. We can find them in many parts of our body, for example, crystalline lens, vitreous body of the eye, and articular cartilage in joints. Their optical, rheological, and tribological functions are closely related to the structure and physical properties of hydrogels as soft matter. The goal of our research program is to discover how living matter achieves the full potential of hydrogels and to develop biomimetic functional materials or materials for tissue replacement on the basis of the science of hydrogels.
Research
Physicochemical Properties of Bio-related Hydrogels
The vitreous body is a tenuous gel that contains collagen and Na-hyaluronate. The fraction of the polymer network is only about 1-2%, and the remaining is water. Therefore, large amount of water is sustained within the dilute polymer network. The vitreous body is located between the lens and the retina that comprises 80% of the overall volume of eye. The functions of the vitreous body are supposed to keep the shape of the eyeball, to absorb the external mechanical shock, to maintain the homoeostasis of the eye, and to regulate the position of the lens. The appearance of fresh vitreous body is transparent, and hence, the vitreous body is considered a uniform tissue. These functions of the vitreous body are still under controversy. The vitreous body has a complex structure, in which the highly flexible Na-hyaluronate is considered to be interwoven by semi-rigid network of collagen threads. Many studies performed to date have suggested that Na-hyaluronate, which has a coil shape, is uniformly distributed throughout the three-dimensional network of collagen fibers that form the triple helix in the vitreous body. However, the structural properties of the vitreous body have not been determined. And essentially no investigations on the dynamics and phase equilibrium properties of the vitreous body, however, have been performed to verify indisputably that the vitreous body is indeed a gel network. Some diseases affect changes in the complex structure of the vitreous body. The collapse of the vitreous body may cause many diseases such as posterior vitreous detachment, vitreous bleeding, and retinal detachment. In the search for the underlying principle of the functions of the vitreous body, it is crucial to understand its physical properties, which leads to promote better understanding of the mechanism of diseases of the vitreous body. From the observations of the dynamics of light scattered by the calf vitreous body, intensity autocorrelation functions that revealed two diffusion coefficients, Dfast and Dslow, were obtained. We developed the theory for describing the density fluctuation of the entities in the vitreous gel system with Na-hyaluronate polymers filled in the meshes of collagen fiber network. The dynamics of collagen and Na-hyaluronate explains two relaxation modes of the fluctuation. The diffusion coefficient of collagen obtained from Dfast and Dslow is very close to that in aqueous solution, which suggests the vitreous body is in the swollen state. The diffusion coefficients were found to be dependent on the position (surface or central part) of the vitreous body from which the scattered light sampled. The inhomogeneous distribution of Na-hyaluronate and collagen and the shell structure of the vitreous body were suggested.
[Figure Caption]
Example of the ultra-structure of the bio-related hydrogel: Schematic diagram of adult vitreous ultra-structure depicting the relationship between hyaluronic acid molecules and collagen fibrils.
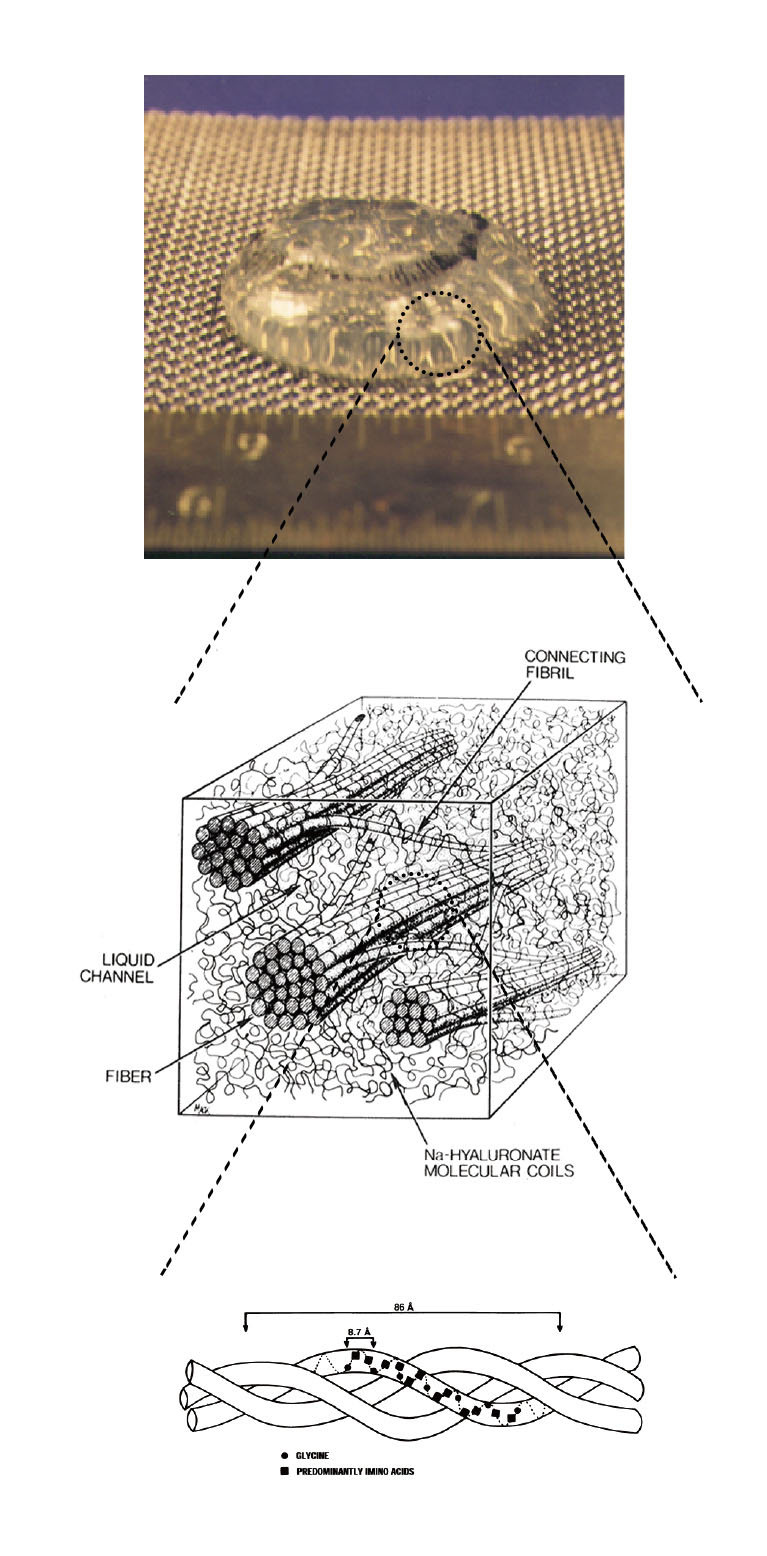
Reference
- [1] T. Matsuura, Y. Hara, F. Taketani, E. Yukawa, S Maruoka, S. Sasaki and M. Annaka: Dynamic Light Scattering Study on Calf Vitreous Body, Macromoleculs, 37, 7784-7790 (2004).
- [2] T. Matsuura, Y. Hara, F. Taketani, E. Yukawa, S Maruoka, K. Kawasaki and M. Annaka: Volume phase transition of bovine vitreous body in vitro and determination of its dynamics, Biomacromoleculs, 5, 1296-1302 (2004).
- [3] M. Annaka, M. Okamoto, T. Matsuura, Y. Hara, S. Sasaki, Dynamic Light Scattering Study of Salt Effect on Phase Behavior of Pig Vitreous Body and Its Microscopic Implication, J. Phys. Chem. B, 111, 8411-8418 (2007).
- [4] Toyoaki Matsuura, Naokazu Idota, Yoshiaki Hara and Masahiko Annaka, Dynamic Light Scattering Study of Pig Vitreous Body, Prog. Colloid and Polym. Sci.,136, 195-204 (2009).
- [5] Masahiko Annaka and Toyoaki Matsuura: Physical Properties of Pig Vitreous Body, in Rheology of Bio-related Soft Matter: Fundamentals and Applications, Isamu Kaneda Ed. Splinger (2016).
Phase Behavior and the Microscopic Structure of the Colloidal Complexes
We investigated the phase behavior and the microscopic structure of the colloidal complexes constituted from neutral/polyelectrolyte diblock copolymers and oppositely charged surfactant by dynamic light scattering (DLS) and small-angle neutron scattering (SANS). The neutral block is poly(N-isopropylacrylamide) (PNIPAm), and the polyelectrolyte block is negatively charged poly(acrylic acid) (PAA). In aqueous solution with neutral pH, PAA behaves as a weak polyelectrolyte, whereas PNIPAm is neutral and in good-solvent condition at ambient temperature, but in poor-solvent condition above ~32°C. This block copolymer, PNIPAm-b-PAA (Fig. 1) with a narrow polydispersity, is studied in aqueous solution with an anionic surfactant, dodecyltrimethylammonium bromide (DTAB, Fig. 1). For a low surfactant-to-polymer charge ratio Z lower than the critical value ZC, the colloidal complexes are single DTAB micelles dressed by a few PNIPAm-b-PAA (Fig. 3a). Above ZC, the colloidal complexes form a core-shell microstructure (Fig. 3b). The core of the complex consists of densely packed DTA+ micelles, most likely connected between them by PAA blocks. The intermicellar distance of the DTA+ micelles is ~ 38 Å, which is independent of the charge ratio Z as well as the temperature. The corona of the complex is constituted from the thermosensitive PNIPAm. At lower temperature the macroscopic phase separation is hindered by the swollen PNIPAm chains. Above the critical temperature TC, the PNIPAm corona collapses leading to form hydrophobic aggregates of the colloidal complexes.
[Figure Captions]
Figure 1 Chemical structures of PNIPAm-b-PAA and DTAB.
Figure 2 Phase diagram obtained for the system of PNIPAm-b-PAA/DTAB/water at c = 0.4 wt% with the degree of neutralization of PAA block α = 0.75.
Figure 3 Structure of PNIPAm-b-PAA/DTAB complexes for (a) Z < ZC and Z > ZC proposed by SANS.
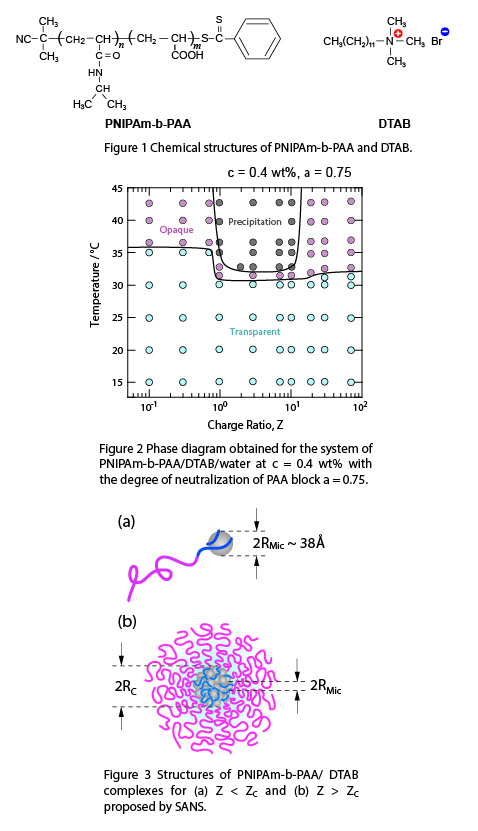
Reference
- [1] M. Annaka, K. Morishita and S. Okabe, Electrostatic Self-Assembly of Neutral/Polyelectrolyte Block Copolymer and Oppositely Charged Surfactant, J. Phys. Chem. B, 111, 11700-11707 (2007).
- [2] Tsuyoshi Matsuda and Masahiko Annaka, Salt Effect on Complex Formation of Neutral/Polyelectrolyte Block Copolymer and Oppositely Charged Surfactant, Langmuir, 24, 5707-5713 (2008).
Dynamic Behavior of hydrophobically modified poly(N-isopropylacrylamides) in water
Among the various families of hydrophobically modified water-soluble polymers, telechelic derivatives bearing hydrophobic end-groups have attracted attention over the decades, primarily because of their ability to form flower-like micellar aggregates which associate above a given polymer concentration, giving rise to highly viscoelastic fluids. Dynamic properties of the telechelic hydrophobically modified poly(N-isopropylacrylamide) (HM-PNIPAM) with an octadecyl group at each chain end are studied by Step strain experiments and dynamic light scattering measurements. HM-PNIPAM forms starlike flower in the dilute regime and develop a fully connected network of flowers above some threshold concentration. Above the percolation threshold, the system is viscoelastic and its dynamics structure consists of two steps for the relaxation of concentration fluctuations: the fast one is dominated by the diffusion but the slow one is almost independent of the wave vector. The terminal time of the stress relaxation τR and the slow time of the dynamic structure factor τS are both presumably controlled by the residence time of an octadecyl group of flower-like micellar aggregate. These results are interpreted according to the so-called two-fluids model, which was initially developed for semidilute polymer solutions and which Appell and coworkers extended to any Maxwell viscoelastic medium characterized by its elastic modulus and terminal time measured by rheology. This model describes consistently and quantitatively the experimental observations.
[Figure Caption]
(a) Chemical structure of HM-PNIPAM (Mw = 20000)
(b)Schematic illustration of micelle network of HM-PNIPAM.
(c) Normalized inverse characteristic times as a function of q2 in reduced unit for 50 - 300 mg mL-1 of HM-PNIPAM at 20°C. The lines are calculated with the values Deff, Dcoll and τR obtained by DLS and rheology measurements. Circle (square) symbols correspond to the slow (fast) mode.
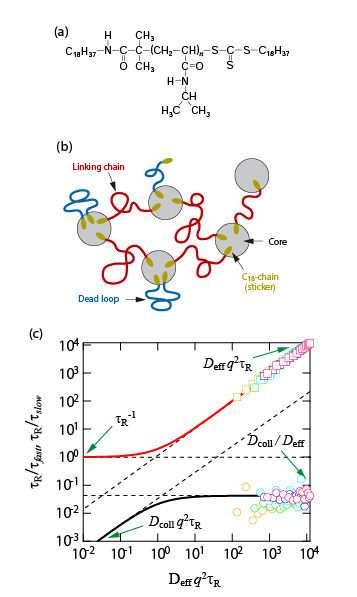
Reference
- [1] T. Fujimoto, E. Yoshimoto and M. Annaka: Study on Self-Assembly of Telechelic Hydrophobically Modified Poly (N-isopropylacrylamide) in Water, Prog. Colloid and Polym. Sci., 136, 77-86 (2009).
A New Biomedical Imaging Technology
The goal of this project is to develop and validate a new biomedical imaging technology based on Brillouin light scattering for non-invasively measuring the biomechanical and rheological properties of tissues, cells, and biomaterials. Spontaneous Brillouin scattering arises from the interaction of light and sound wave that is inherently present in a sample. By detecting the spectral shifts in the scattered light, the sample’s hypersonic viscoelastic properties can be measured at microscopic spatial resolution without physical contact. Brillouin spectroscopy has long been known in material physics and environmental sensing. However, its potential applications in biological sciences and clinical medicine have not been fully explored. We aim to develop new practical instruments and validate them for a number of potential applications. Brillouin imaging has a potential to be a useful diagnostic tool in ophthalmology for early detection and screening of various lens and corneal problems, such as presbyopia, cataracts, and corneal ectasia. This technology may also be useful in the study of cellular biomechanics in cancer and tissue engineering.
[Figure Caption]
(A) Accommodation is the process by which the vertebrate eye changes optical power to maintain a clear image or focus on an object as its distance varies.
(B) Left: Schematic of the murine eye. Right: images of the crystalline lens extracted after measurement. The arrow indicates the beam entrance direction.
(C) In situ characterization of the crystalline lens in a mouse eye. Brillouin frequency shifts measured at various depths along the central optic axis (blue circles), showing a twofold increase from the outer layers (cortices) towards the lens centre (nucleus). Error bars represent the measurement uncertainty. C: cornea, A: aqueous humour, L: lens, V: vitreous humour, and R: retina.
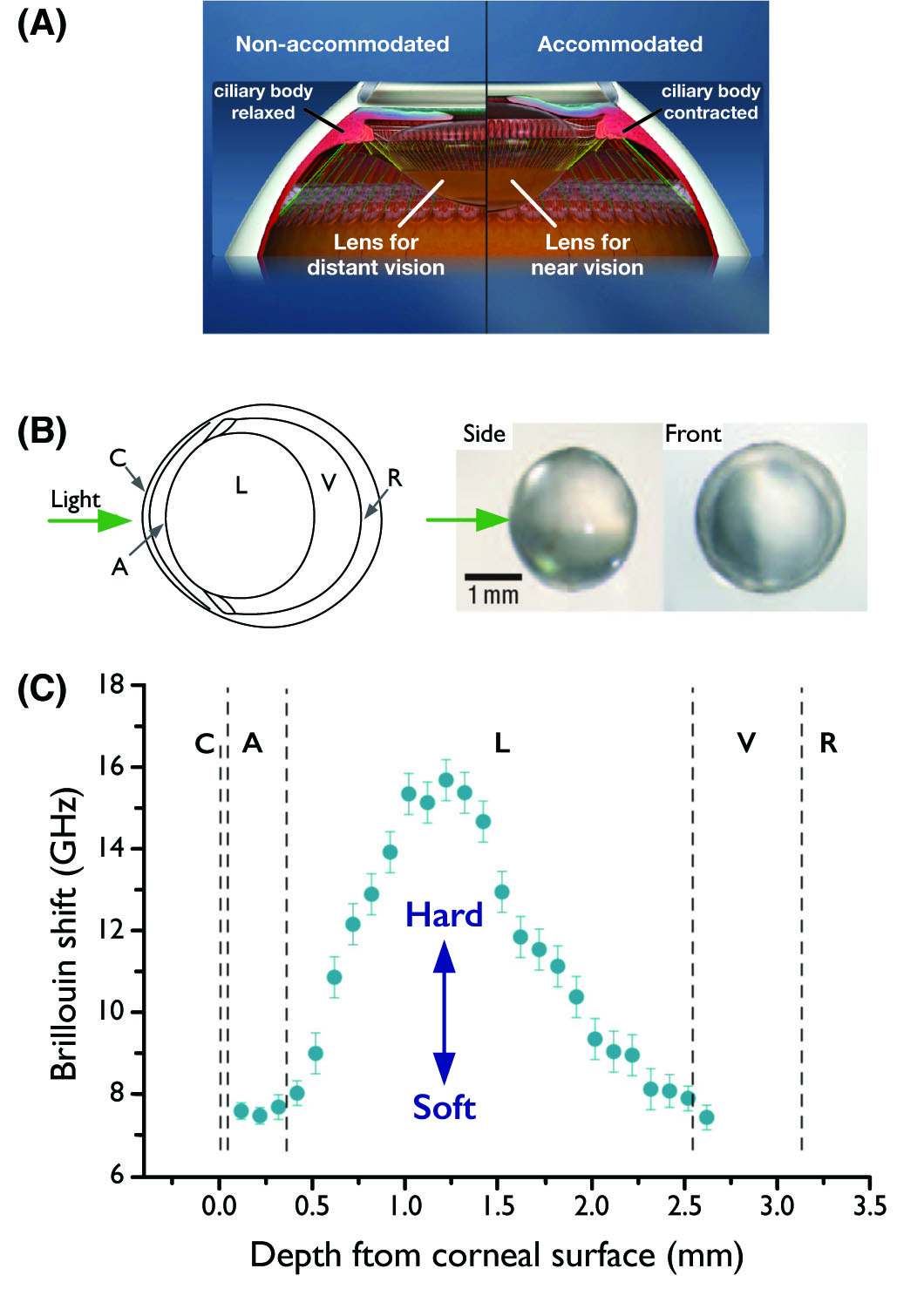
Biomaterials for Ophthalmic Use
Cataracts are caused by optical defects of the natural lens that develop with age and can lead to increasingly blurred vision and blindness. Currently, plastic lenses can be used to surgically replace the natural lens but they are not a perfect replacement, for example they tend to be mono-focal, limiting the eye’s ability to focus outside a set range. They are also not a proper fit, which can cause problems such as misalignment. We developed a nanocomposite material that is injected into the capsular bag left behind once the affected lens has been removed. The nanocomposite – an aqueous suspension of silica nanoparticles that also contains hydrophobically-modified polyethylene glycol – fills the capsular bag and then sets into a gel at body temperature, fitting the cavity snugly and eliminating the need for complicated surgery. With mono-focal lenses, you need to choose your focus: very distant or very near. ‘In our case, the gel itself accommodates focus on the retina. Although injectable lenses have been explored before, previous attempts involved injecting a monomer solution, which then polymerized in situ. This raised concerns about the toxicity of unreacted monomer and the lengthy polymerization process resulting in leakage of the monomer. Although injectable gels that solidify and form a viable structure in the body are very exciting,
[Figure Caption]
(a) Injection of E30S30 nanocomposite gel into the pig lens capsular bag by using syringe with 27-gague needle.
(b) Lens capsule filled with E30S30 nanocomposite gel (top view).
(c) Lens capsule filled with E30S30 nanocomposite gel (side view). E30S30 nanocomposite gel is confirmed to form a robust gel rapidly and keep a proper shape in the pig lens capsular bag.
(d) Objects viewed though the in-situ endocapsular gelled E30S30 lens appeared clear and undistorted. E30S30 lens provides same magnification as it is for the dissected natural pig lens. Dissected natural pig lens is shown on the downside for comparison.
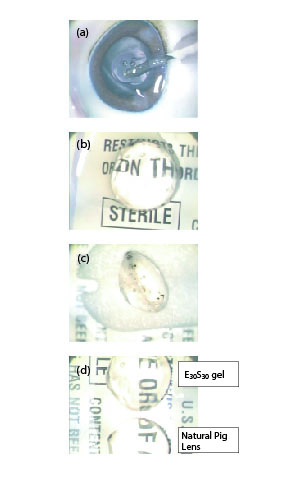
Reference
- [1] S. Maruoka, T. Matsuura, Y. Hara, M. Kodama and M. Annka: Biocompatibility of polyvinylalchol gel as a vitreous substitute: Experimental Eye Research, 31, 599-606 (2006).
- [2] Masahiko Annaka, Kell Mortensen, Martin E. Vigild, Toyoaki Matsuura, Souichiro Tsuji, Tetsuo Ueda, Hiroki Tsujinaka: Design of an Injectable in Situ Gelation Biomaterials for Vitreous Substitute, Biomacromolecules, 12, 4011-4021 (2012).
- [3] Masahiko Annaka, Kell Mortrensen, Toyoaki Matsuura, Masaya Ito, Katsunori Nochioka, Nahoko Ogata: Organic–inorganic nanocomposite gels as an in situ gelation biomaterial for injectable accommodative intraocular lens, Soft Matter, 8, 7185-7196 (2012).
Introducing molecular orientation and designed microstructure into hydrogels
Hydrogels prepared in the usual manner are macroscopically isotropic and uniform. Biological gels in living matter, on the other hand, often shows anisotropy due to the molecular orientation and fine structure at a micrometer scale. We found that the anisotropic molecular orientation and macroscopic pattern formation could be induced in hydrogels when the gelation occurred under the condition that crosslinkers diffused into polymer solutions, and investigated such characteristic gel structures [1]. We also have developed a method to obtain mechanically tough hydrogel films with a designed substructure of several-hundred-microns of which rigidity, swellability, and a thermoresponsive property are different from the bulk by applying a certain photolithographic technique in the preparation process of polymer-clay nanocomposite gels [2,3].
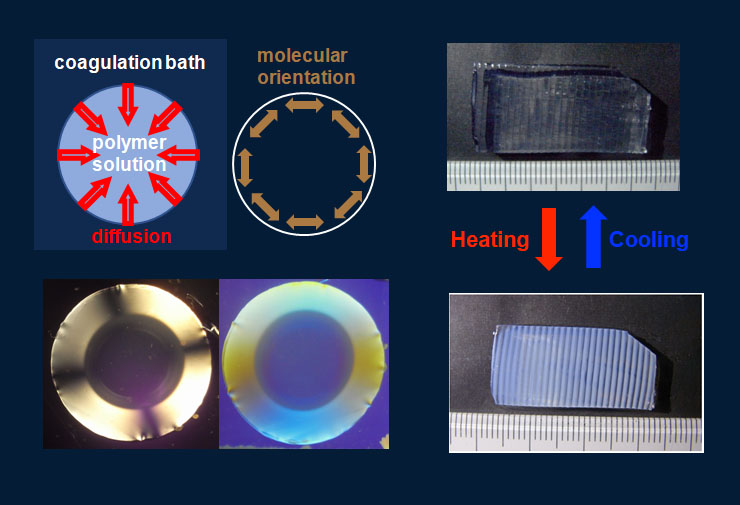
Reference
- [1] Yasuyuki Maki, Kazuya Furusawa, Takao Yamamoto, Toshiaki Dobashi, Structure formation in biopolymer gels induced by diffusion of gelling factors, J. Biorheol.32, 27-38 (2018).
- [2] Yasuyuki Maki, Poly(N,N-dimethylacrylamide)-clay nanocomposite hydrogels with patterned mechanical properties, Colloid Polym. Sci. 297, 587-594 (2019).
- [3] Yasuyuki Maki, Toshiaki Dobashi, Poly (N-isopropylacrylamide)-Clay Nanocomposite Hydrogels with Patterned Thermo-Responsive Behavior, Trans. MRS-J 42, 119-122 (2017).
Lubrication and Friction of Hydrogels
The biological tissues have very low friction, as we can easily see from the fact that we do not feel any friction resistance when we move our body.
A hydrogel is soft and contains much water, so it can be said to have properties close to living tissues and is drawing attention as a model substance for living tissue and as a substituting material for medical treatment. We found that the sliding friction changes when the μm order surface geometry was given to polyvinyl alcohol gel. The random surface roughness increased the friction of hydrogels and the surface dimples decreased it. These findings are expected to advance understanding of changes in the surface geometry of articular cartilages due to diseases and to promote the application of hydrogel to artificial articular cartilage.
Left: Surface image of sliding hydrogels with surface dimples
Right: Dependence of friction stress with sliding velocity of hydrogels with surface dimples
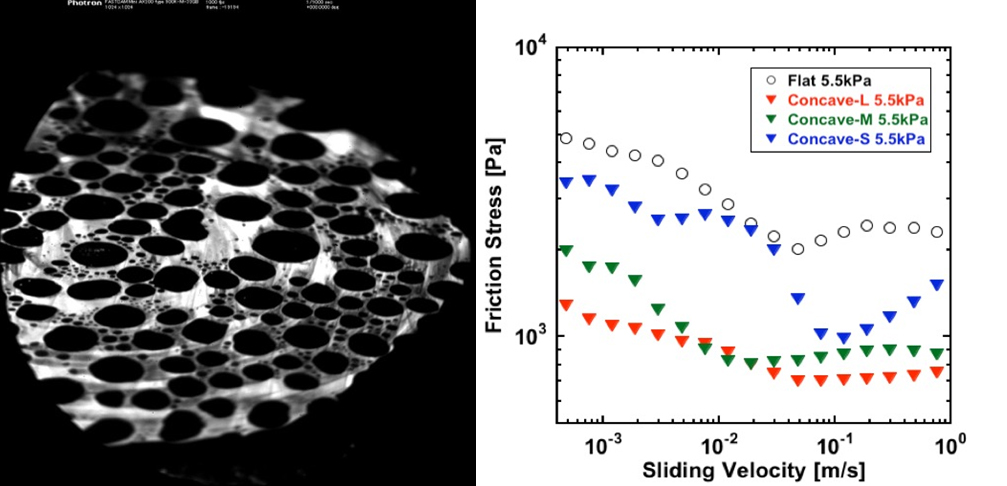
Reference
- S. Yashima, N. Takase, T. Kurokawa, J. P. Gong, Friction of hydrogels with controlled surface roughness on solid flat substrates, Soft Matter 10, 3192–3199 (2014).
- S. Yashima, V. Romero, E. Wandersman, C. Frétigny, M. K. Chaudhury, A. Chateauminois, A. M. Prevost, Normal contact and friction of rubber with model randomly rough surfaces, Soft Matter 11, 871-881 (2015).